Abstract
The costs associated with the treatment of medical device and surgical site infections are a major cause of concern in the global healthcare system. To prevent transmission of such infections, a prophylactic surface system that provides protracted release of antibacterial silver ions using low intensity direct electric current (LIDC; 28 μA system current at 6 V) activation has been recently developed. To ensure the safety for future in vivo studies and potential clinical applications, this study assessed the biocompatibility of the LIDC-activated interdigitated silver electrodes-based surface system; in vitro toxicity to human epidermal keratinocytes, human dermal fibroblasts, and normal human osteoblasts, and antibacterial efficacy against Staphylococcus aureus and Escherichia coli was evaluated. The study concluded that the technological applications of the surface system for medical devices and surgical tools, which contact human tissues for less than 1.5 h, are expected to be self-sterilizing without causing toxicity in vivo.





Similar content being viewed by others
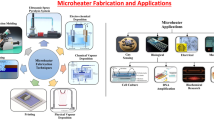
References
Edwards JR, Peterson KD, Mu Y, Banerjee S, Allen-Bridson K, Morrell G, Dudeck MA, Pollock DA, Horan TC. National Healthcare Safety Network (NHSN) report: data summary for 2006 through 2008. Am J Infect Control. 2009;37:783–805.
CDC. National Hospital Discharge Survey: number, rate, and standard error of all-listed surgical and nonsurgical procedures for discharges from short-stay hospitals, by selected procedure. National Center for Health Statistics. 2009. p. 1–3.
Hall MJ, Owings MF. National Hospital Discharge Survey. Advance Data from Vital and Health Statistics. United States: Centers for Disease Control and Prevention (CDC); 2000. p. 1–19.
Ziegler-Graham K, et al. Estimating the prevalence of limb loss in the United States: 2005 to 2050. Arch Phys Med Rehab. 2008;89:422–9.
Del Pozo JL, Patel R. Infection associated with prosthetic joints. N Engl J Med. 2009;361:787–94.
Whitehouse JD, Friedman ND, Kirkland KB, Richardson WJ, Sexton DJ. The impact of surgical-site infections following orthopedic surgery at a community hospital and a university hospital: adverse quality of life, excess length of stay, and extra cost. Infect Cont Hosp Epidemiol. 2002;23(4):183–9.
AAOS. Orthopaedic infection prevention and control: an emerging new paradigm. American Academy of Orthopaedic Surgeons. Proceedings from 77th annual meeting. 2010;1–6.
Cabrita H, Croci A, De Camargo O, De Lima A. Prospective study of the treatment of infected hip arthroplasties with or without the use of an antibiotic-loaded spacer. Clinics (Sao Paulo). 2007;62:99–108.
Tu J, Yu M, Lu Y, Cheng K, Weng W, Lin J, Wang H, Du P, Han G. Preparation and antibiotic drug release of mineralized collagen coatings on titanium. J Mater Sci-Mater M. 2012; doi:10.1007/s10856-012-4692-5.
Samberg ME, Monteiro-Riviere NA. In vitro and in vivo toxicity of silver nanoparticles. In: Bhushan B, editor. Encyclopedia of nanotechnology, vol. 10. Heidelberg: Springer; 2012. p. 1069–77.
Lok CN, Ho CM, Chen R, He QY, Yu WY, Sun H, Tam PK, Chiu JF, Chen CM. Proteomic analysis of the mode of antibacterial action of silver nanoparticles. J Proteome Res. 2006;5:916–24.
Panácek A, Kvítek L, Prucek R, Kolář M, Veceřová R, Pizúrova N, Sharma V, Nevěcná T, Zbořil R. Silver colloid nanoparticles: synthesis, characterization, and their antimicrobial activity. J Phys Chem B. 2006;110:16248–53.
Samberg ME, Orndorff PE, Monteiro-Riviere NA. Antibacterial efficacy of silver nanoparticles of different sizes, surface conditions and synthesis methods. Nanotoxicology. 2011;5(2):244–53.
Bologna RA, Tu LM, Polansky M, Fraimow HD, Gordon DA, Whitmore KE. Hydrogel/silver ion-coated urinary catheter reduces nosocomial urinary tract infection rates in intensive care unit patients: a multicenter study. Urology. 1999;54(6):982–7.
Massè A, Bruno A, Bosetti M, Biasibetti A, Cannas M, Gallinaro P. Prevention of pin track infection in external fixation with silver coated pins: clinical and microbiological results. J Biomed Mater Res. 2000;5(53):600–4.
Sheehan E, McKenna J, Mulhall KJ, Marks P, McCormack D. Adhesion of Staphylococcus to orthopaedic metals, an in vivo study. J Orthop Res. 2004;22:39–43.
Fuller T, Wysk R, Charumani C, Kennett M, Sebastiennelli W, Abrahams R, Shirwaiker RA, Voigt R, Royer P. Developing an engineered antimicrobial/prophylactic system using electrically activated bactericidal metals. J Mater Sci-Mater M. 2010;21(7):2103–14.
Singh R, Singh D. Radiation synthesis of PVP/alginate hydrogel containing nanosilver as wound dressing. J Mater Sci-Mater M. 2012; doi:10.1007/s10856-012-4730-3.
Shirwaiker RA, Wysk RA, Kariyawasam S, Carrion H, Voigt RC. Micro-scale fabrication and characterization of a silver–polymer based electrically activated antibacterial surface. Biofabrication. 2011;3(1):015003.
Shirwaiker RA. The characterization of the antibacterial efficacy of an electrically activated silver ion-based surface system. University Park: The Pennsylvania State University; 2011.
Hussain SM, Hess KL, Gearhart JM, Geiss KT, Schlager JJ. In vitro toxicity of nanoparticles in BRL 3A rat liver cells. Toxicol In Vitro. 2005;19(7):975–83.
Kim S, Choi JE, Choi J, Chung K-H, Park K, Yi J, Ryu D-Y. Oxidative stress-dependent toxicity of silver nanoparticles in human hepatoma cells. Toxicol In Vitro. 2009;23(6):1076–84.
Park E-J, Yi J, Kim Y, Choi K, Park K. Silver nanoparticles induce cytotoxicity by a Trojan-horse type mechanism. Toxicol In Vitro. 2010;24(3):872–8.
Samberg ME, Oldenburg SJ, Monteiro-Riviere NA. Evaluation of silver nanoparticle toxicity in skin in vivo and keratinocytes in vitro. Environ Health Perspect. 2010;118:407–13.
Acknowledgments
The cytotoxicity evaluation experiments were supported by a research Grant from ArgentumCidalElectrics, Inc., Pittsburgh, PA. The antimicrobial efficacy testing experiments were supported by a research Grant from North Carolina State University’s Research and Innovation Seeding Funding (RISF) Program.
Conflict of interest
The authors declare that they have no conflict of interest.
Author information
Authors and Affiliations
Corresponding author
Rights and permissions
About this article
Cite this article
Samberg, M.E., Tan, Z., Monteiro-Riviere, N.A. et al. Biocompatibility analysis of an electrically-activated silver-based antibacterial surface system for medical device applications. J Mater Sci: Mater Med 24, 755–760 (2013). https://doi.org/10.1007/s10856-012-4838-5
Received:
Accepted:
Published:
Issue Date:
DOI: https://doi.org/10.1007/s10856-012-4838-5